- Investigations on the mechanisms of drought tolerance are a prerequisite for creating a science-based strategy for improving the resistance of crop plants under conditions of global climate change. Resurrection plants are an excellent model for studying the specific protective mechanisms for the survival of plant species during desiccation to air-dry state. Of particular importance for elucidating the survival strategy of the resurrection plant Haberlea rhodopensis is the study of defense mechanisms during the first hours of recovery after complete desiccation. It was found that the changes in the structural and functional characteristics of thylakoid membranes, such as increased fluidity of the lipid phase, increased abundance of protective proteins, enhancement of alternative cyclic electron pathways are crucial for the restoration of photosynthetic function during the first hours of rehydration. The maintenance of a high antioxidant activity (enzymatic and non-enzymatic) and upregulation of individual enzyme isoforms indicated their essential role in protecting plants from oxidative damage during the onset of recovery (Mihailova et al., Plants 11(17): 2185, 2023; Georgieva et al., Photosynthetica 60(1): 136-146, 2023; Mihailova et al., Plants 11(2): 175, 2023).
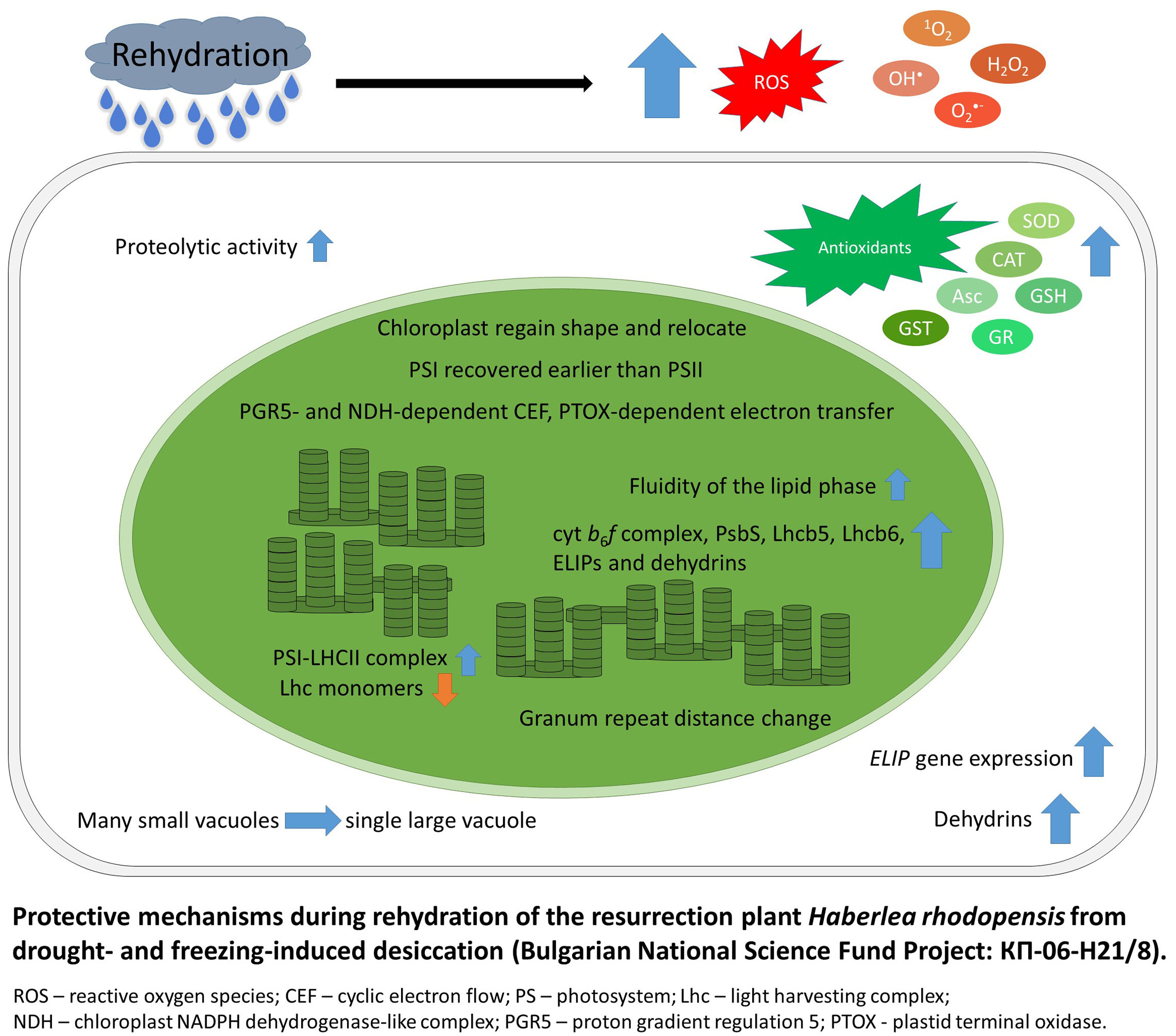
- A new approach for quantitative and qualitative proteomics based on stable isotope-coded protein labeling (ICPL) in combination with polyacrylamide gel electrophoresis (PAGE) and liquid chromatography tandem mass spectrometry (LC-MS/MS) was used to elucidate the changes in chloroplast proteome of isoprene-emitting and non-isoprene emitting poplars. It was demonstrated that the suppression of isoprene synthase by RNA interference, leading to the development of non-isoprene emitting poplar genotypes, decreased the levels of chloroplast proteins involved in photosynthesis and increased levels of histones and ribosomal proteins. The removal of isoprene emission in poplar caused large changes in the proteome of chloroplasts affecting the structural organization of thylakoid membranes. The absence of isoprene synthase activity and consequently of isoprene emission led to down-regulation of proteins related to the light reactions of photosynthesis, redox regulation and oxidative stress defense (Velikova et al., Journal of Proteome Research 13: 2005-2018, 2014).
- Isoprene plays a crucial role in preserving the intactness of thylakoid membranes under heat stress, and more specifically, the functionality of photosystem II, suggesting some modifications in the lipid environment of thylakoid membranes (Velikova et al., Plant Physiology 157: 905-916, 2011).
- For the first time, it was proven that the removal of isoprene emission in poplar causes large changes in the lipid content affecting the structural organization of thylakoid membranes. It is demonstrated that the total amount of monogalactosyldiacylglycerols, digalactosyldiacylglycerols and phospholipids is reduced in chloroplasts when isoprene emission is blocked. The chloroplasts of non-isoprene emitting poplar are more rounded and contain fewer grana stacks and longer stroma thylakoids, more plastoglobules, and larger associative zones between chloroplasts and mitochondria (Velikova et al., Plant Physiology 168: 859-870, 2015).
- Endogenous isoprene has an important protective role in plants by regulating the level of reactive oxygen and nitrogen species (Loreto and Velikova, Plant Physiology 127: 1781-1787, 2001; Velikova et al., Physiologia Plantarum 122: 219-225, 2004; Velikova et al., New Phytologist 166: 419-426, 2005; Velikova et al., Plant Signaling & Behavior 7: 139-141, 2012; Vanzo et al., Plant Physiology 170: 1945-1961, 2016).
- The unchanged chlorophyll content and amounts of chlorophyll–protein complexes, together with reversible modifications in PSII electron transport and enhanced probability for non-radiative energy dissipation as well as increased polyphenolic synthesis and high antioxidant activity during desiccation of Haberlea rhodopensis contribute to its drought resistance and fast recovery after rehydration. The increased sucrose content and activation of xanthophyll cycle are important defense mechanisms during desiccation. In addition, secondary vacuoles are formed peripherally in the cell, and they grow by accumulating the soluble sugars, which originate from starch degradation. (Georgieva et al., Photosynthesis Research 85: 191-203, 2005; Georgieva et al., Planta 225: 955-964, 2007; Georgieva et al., Protoplasma 254(1): 193-201, 2017).
- The desiccation tolerance of H. rhodopensis was investigated under high temperature. Regardless of the stronger membrane damage and inhibition of the photosynthetic activity upon dehydration at 38°С compared to dehydration at 23°С as well as the slower recovery after rehydration, plants fully restored their physiological activity (Mihailova et al., 2009, Biotechnology and Biotechnological equipment, Special issue, 23 (2): 561-564; Mihailova et al., 2011, Photosynthesis Research 108: 5-13; Velitchkova et al., 2013, Photosynthetica 51(4): 630-640).
- Two different ecotypes of H. rhodopensis adapted to low and high light intensity in natural habitats were used to study the effect of light during desiccation. In contrast to the great differences in the morphology of plants, the chloroplast ultrastructure and the organization of thylakoids were similar in both ecotypes. Desiccation induced some PSII core degradation, stronger in sun plants, and decreased the LHCII antenna size of both photosystems. Monomerization of antennae was observed in shade plants, while the proportion of trimeric LHCII was constant in sun plants. Plants from both ecotypes used different mechanisms for quenching the excess excitation energy – shade plants were mainly protected by thermal re-emission by photoinactivated PSII reaction centers, while sun plants relied more on the quenching mechanism in the light-harvesting antennae (Sarvari et al., Journal of Plant Physiology 171 (17): 1591-1600, 2014; Solti et al., Journal of Photochemistry and Photobiology B: Biology 130: 217-225, 2014; Rapparini et al., Environmental and Experimental Botany 113: 67-79, 2015).
- For the first time the polyphenol profiles (fingerprints) of the resurrection plant Haberlea rhodopensis are obtained. The polyphenol complex of H. rhodopensis includes only two types of glycosides – phenylethanoid glucosides and hispidulin 8-C-glucosides. The phenylethanoid glucosides (myconoside and paucifloside) play the main role in the protection of H. rhodopensis during desiccation, preserving the membranes against free radical-induced oxidation. The significant enhancement of the antioxidant capacity at 8% RWC corresponded to an enormous increase in flavonoid content. The content of investigated antioxidants and the activity of antioxidant enzymes increased upon desiccation of Haberlea rhodopensis, reaching a maximum in air-dry state, which is a distinguishing feature in resurrection plants. These antioxidants can be utilized during the early stages of rehydration, thus helping for the recovery of plants. Besides drought was the main factor in regulation for most of the genes examined, some were light modulated – like genes coding for putative superoxide dismutase (SOD), ascorbate peroxidase (APX) and thioredoxin (TRX). Some of the investigated genes retained their enhanced expression longer during recovery, indicating their importance for survival and recovery in sun plants. A newly annotated gene, ELIP, showed similarity to protein that encodes early light-induced protein (ELIP) from another resurrection plant Boea hygrometrica, Gesneriaceae. NA1 gives a homology to LEA1 of B. hygrometrica and PTL to a chloroplastic transporter protein of the outer envelope membrane, OEP16 from A. thaliana. (Mihailova et al. 2017, Plant Molecular Biology Reporter, 35 (3): 313-322, 2017; Georgieva et al., Plant Physiology and Biochemistry 114: 51-59, 2017).
- For the first time, the high resistance of H. rhodopensis to freezing temperatures has been proven. Temperatures below -6 ° C induce desiccation of plants and they survive the harsh winter conditions in a dry state. It was found that ultrastructural changes in mesophyll cells, reversible downregulation of photosynthesis, readjustments in the abundance/ organization of the pigment protein complexes and the main photosynthetic proteins together with increased thermal energy dissipation during chilling and freezing temperatures enable plants to survive harsh winter conditions (Mihailova et al., Environmental and Experimental Botany 178: 104157, 2020). The slow water uptake during the initial hours of rehydration prevents cellular damages. The role of PSII reaction center quenching during the recovery was suggested. During the first hours of rehydration, prominent alterations in energy transfer between photosynthetic complexes occurred. PSI activity recovered faster compared to PSII, which may be due to the activation of alternative electron flows to PSI (Georgieva et al., Photosynthetica 58: 911-921, 2020).
- An unusual high temperature of thermoluminescence B band registered in leaves of the resurrection plant H. rhodopensis was observed. Progressive dehydration of the leaves did not shift the B band temperature maximum position, which is an indication of more stable stored S2/3QB– charge pairs, enabling the successful charge separation in desiccated leaves (Peeva and Maslenkova, Plant Biology 6: 319-324, 2004). These thermoluminescence characteristics were also observed in isolated thylakoid membranes from fully hydrated and desiccated H. rhodopensis leaves, showing that the stable charge separation represents an intrinsic property of PSII electron transport and is associated with desiccation tolerance of this homoiochlorophyllous plant (Maslenkova et al., Handbook of Plant and Crop Stress, Third Edition, 435-446, 2010).
- Leaf net CO2 uptake of the resurrection plant H. rhodopensis shows a low rate under saturating photosynthetic photon flux densities in air, due to the limitations for CO2 from ambient air to the chloroplasts. The drought induced decrease of photosynthesis is diffusion limited by both stomatal closure and mesophyll conductance for CO2. Primary photosynthetic reactions are resistant to drought (Peeva and Cornic, Environmental and Experimental Botany 65: 310-318, 2009).
- Thermoluminescence and P700 redox kinetics are complementary tools to investigate the cyclic/chlororespiratory electron pathways in barley leaves under stress conditions. The fast P700+ reduction phase corresponds to the ferredoxin plastoquinone reductase pathway, triggered by an overreduction of the PSI acceptor pool. The afterglow thermoluminescence band provides specific information on the NDH pathway induced in order to provide additional ATP by CEF1, reflected also by acceleration of the slow P700+ reduction phases (Peeva et al., Physiologia Plantarum 144: 83-97, 2012).
- The main lipid classes and their fatty acid composition in different halophytes (Bassia hirsute Aschers, Euphorbia peplis L., Salicornia europeae L., Calystegia soldanella, Calistegia sepium, Suaeda maritime, Stachys maritime) was investigated. The results indicated that salinity resistance correlated with regrouping of the main lipid classes, changes in their fatty acid profiles as well as in the 18:2/18:3 ratio (linoleic/linolenic acid) (Ivanova et al., Compt. rend. Acad. bulg. Sci. 53: 83-86, 2000; Ivanova et al., Z. Naturforsch. 58c: 783-788, 2003; Ivanova et al., Compt. rend. Acad. bulg. Sci. 58(7): 765-770, 2005; Ivanova et al., General and Applied Plant Physiology (Bulg.) SI: 125-130, 2006; Ivanova et al., Nat. Prod. Res. 23: 448-454, 2009; Косаковская и др., Plant Introduction (Укр.) 1: 72-77, 2012).
- Tribulus terrestris is a medicinal plant with significant pharmacological properties. The comparative analysis of T. terrestris plants grown on soils with different salinity showed that soil salinity affected significantly lipid composition, membrane permeability and the content of furostanol and spirostanol saponins (Ivanova et al., Compt. Rend. Acad. Bulg. Sci. 61: 55-62, 2008; Georgiev et al., Biotechnol. and Biotechnol. EQ. 24: 49-52, 2010).